The eyes (vision), in their diversity of form and structure, allow any organism (invertebrates and vertebrates) to collect light energy (photons), convert it into electrical energy which will then be transmitted to the brain via neurons where it will be analyzed and interpreted to give birth to a mental representation of the environment to the animal allowing it to move, locate prey or a sexual partner.
However, the eye of arthropods is different from that of vertebrates both in its anatomy and its mode of functioning. This series of three articles attempts to provide a general synthesis on vision in insects: 1) Anatomy and structure of the eyes ; 2) Vision and perception of movement ; 3) Physiology of receptors and mechanisms regulating sensitivity to light.
I. Transduction of light energy into electrical energy
The conversion of a light signal into electrical energy and then into a nervous message involves a mechanism called phototransduction. This process involves photosensitive protein receptors: visual pigments, called rhodopsins. In insects, these proteins are found in small specialized structures: the chromophores, they themselves present in the rhabdomere ommatidies (read ce article).
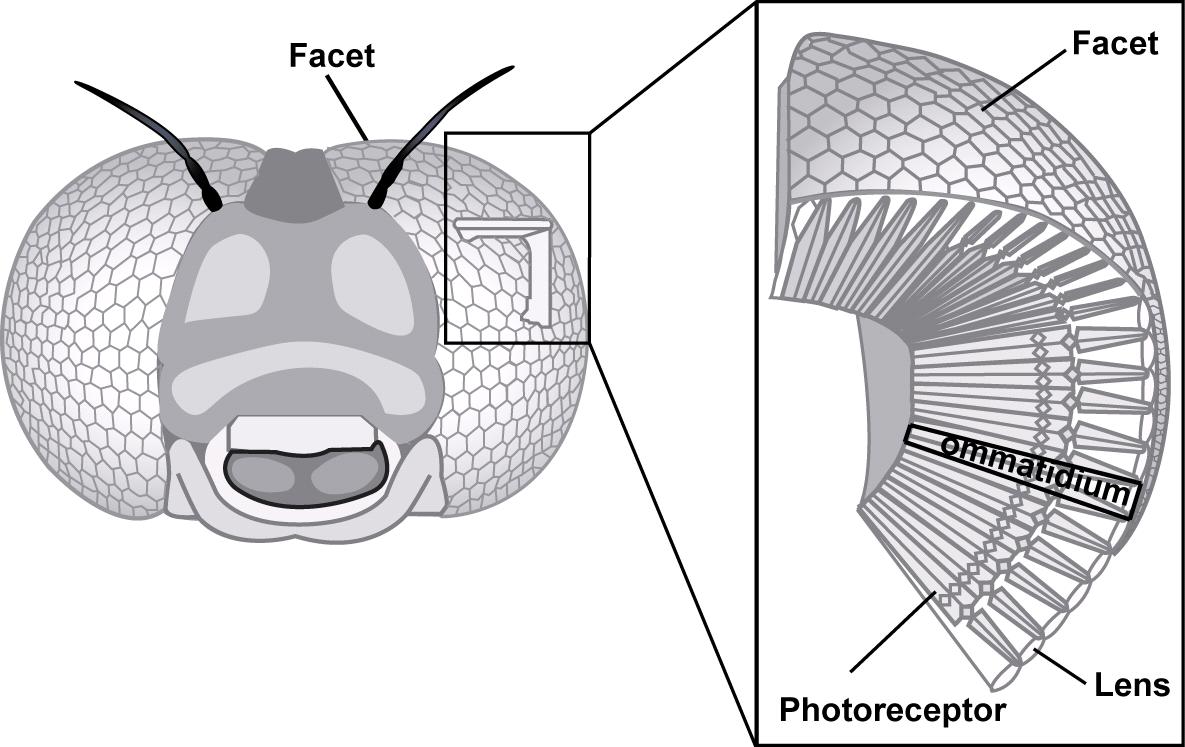
For example, in Drosophila melanogaster (Drosophila melanogaster), 500 to 2000 rhodopsin molecules are present in each of the 40 000 microvilli contained in a rhabdomere of an ommatidie.
When a photon (light particle) is absorbed by a rhodopsin molecule, a complex cascade of chemical events causes a depolarization (change in electrical charge) of the membrane of a nerve cell (neuron).
The probability for a photon to encounter a rhodopsin molecule depends on the length of the rhabdomere : thus, with a length of 80µm, the rhabdomere of the drosophila absorbs 26% of the light against 100% in the dragonfly. In other species, nocturnal or in the shadows, a tapetum* (reflecting surface) reflects light back a second time towards the rhabdomere, thus increasing the probability of a photon meeting a rhodopsin molecule and thus the sensitivity of the visual organ to light.
*Tapetum : the same principle is found in the eyes of nocturnal vertebrates like cats, their eyes reflecting the light from the headlights of a car for example.
II. Adaptation to changes in brightness
Nycthemeral cycles cause large fluctuations in luminosity between day and night : 4×10.20 photons per square meter per second during the day against 10.10 photons during the night (variation of a factor of 40 billion!). In order to overcome this environmental constraint, insects have developed multiple adaptations located at two levels: 1) Regulation of the quantity of light reaching the photoreceptors; 2) Modification of the sensitivity of the photoreceptors.
II.1. Regulation of the amount of light reaching the photoreceptors
The amount of light that reaches the rhabdomere is regulated by the movement of pigments in the cells, movement sometimes associated with the anatomical modification of ommatidies. This adaptation is mostly found in eyes composed of “superposition” type (lire cet article) (Illustration 1 below).
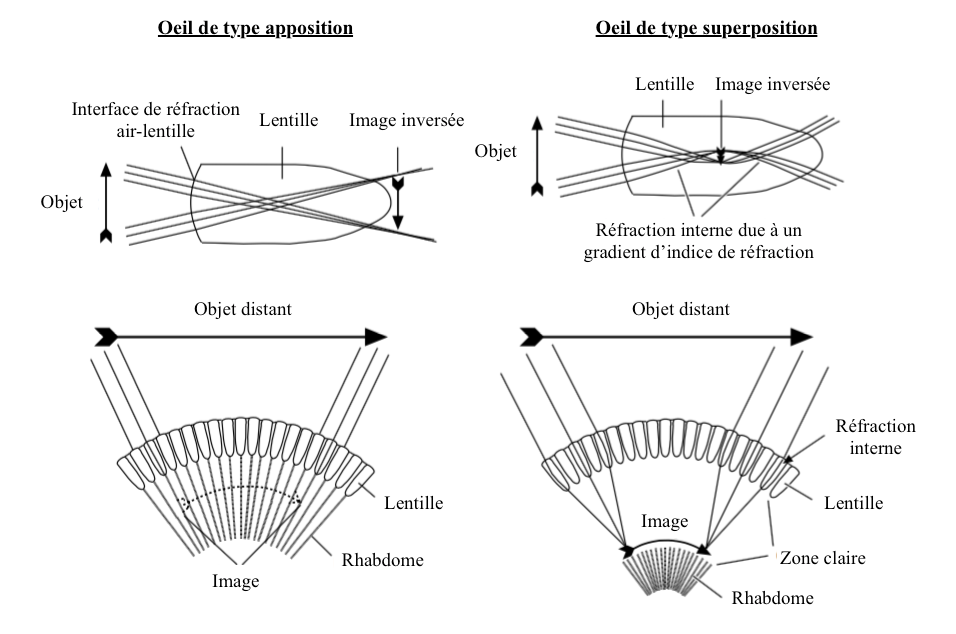
- Yeux de types superposposition
Adaptations are at two levels:
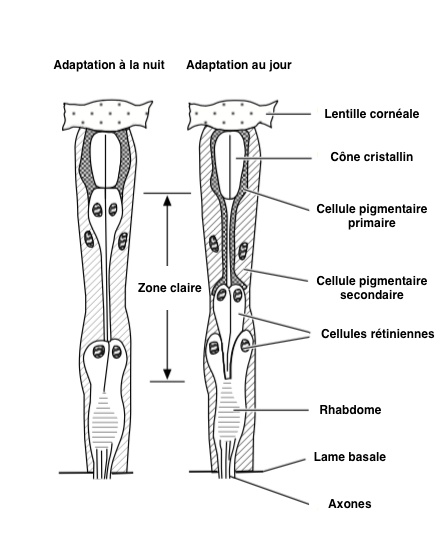
– Pigments : in a dark environment, pigments are located in the distal area of pigment cells, allowing photons to propagate between ommatidies : clear area (Illustration 2 opposite – click on to enlarge). Conversely, in the presence of high light intensity, the pigments move towards the centre of the cells in order to limit the entry of light by reducing the quantity of photons entering the ommatidie. At this moment, the functioning of the eye is similar to that of the “apposition” type, where each ommatidie functions independently of the others (lire this article).
A similar process exists in our eyes, the lens closes or opens more or less depending on the light intensity present in the environment.
– Photoreceptor cells : adaptation to the change in luminosity is achieved by the extension and compression of the crystalline cone, confining the pigment to the peripheral parts of the ommatidia. In high light conditions, the photoreceptor cells are short and the primary pigment cells extend under the lens, these displacements can represent nearly 15µm.
Adjusting to changing light conditions can take several minutes or more. In ants Camponotus, the first changes occur 15 minutes after a change in light intensity to end 2 hours later. There is a wide variety of tempo between species. Thus, in moths of the genus Cydia (Carpocapses), the complete process, lasting 1 hour, begins 1h30 before dusk or dawn so that the adaptation is completed 30 min before the total change in luminosity.
The perceived light intensity is also dependent on the habitat in which the insect evolves. For example, the cave beetle of the genus Zopholas perceives a magnitude brightness 5 times lower than diurnal species of the same family (Tenebrionidae), such as the genus Tenebrio.
- Apposition eyes
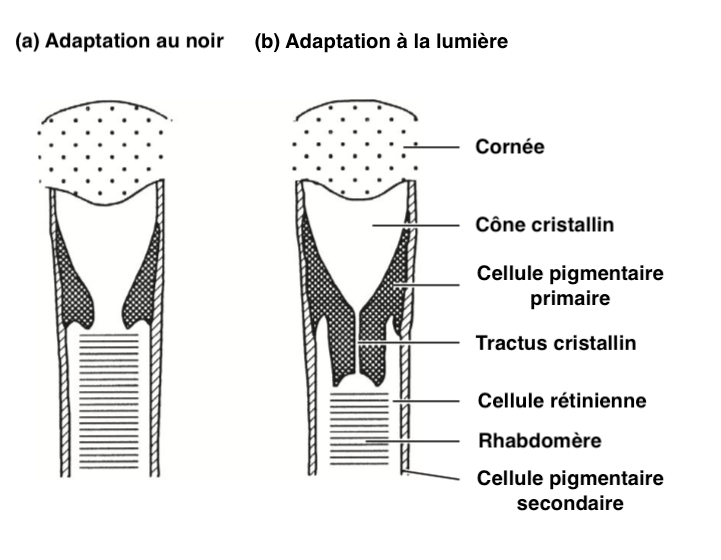
Pigment movements comparable to eyes of the superposition type are observed in those of the apposition type.
For example, in ants of the genus Camponotus, primary pigment cells contract when the brightness increases, compressing the crystalline cone, forming a narrow crystalline tract surrounded by primary pigment cells (Illustration 3 opposite). This narrowing decreases the entry of light and only the light arriving in the axis of the ommatidie reaches the rhabdomere. Each ommatidie is regulated independently of the others.
II.2. Modification of photoreceptor sensitivity
Receptor sensitization is regulated in apposition-type eyes by a structural change in photoreceptor cells. Their structure varies according to the light availability absorbed by rhodopsin molecules.
Absorbent pigment granules are present inside the receptor cells surrounding the interior of the rhabdomere microvilli (Illustration 4 below). In clarity, the pigment granules migrate towards the inner surface of the rhabdomere and absorb light circulating along it, thus reducing the amount of light and light intensity received by the photoreceptors. In the dark, the process is reversed: the absorbent pigments migrate towards the periphery.
The movements of these pigments, contrary to those located in pigment cells, are as for them fast : a few seconds hardly are enough for example in diptera (flies) and lepidoptera (butterflies).
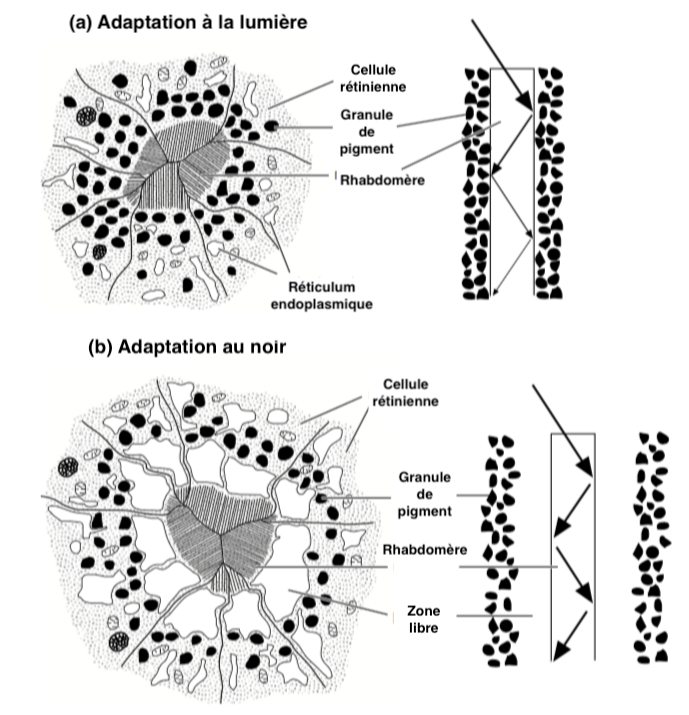
III. Spectral sensitivity and chromatic vision
Photoreceptor cells have a sensitivity range (Gaussian profile) at wavelengths between 330 and 640 nm (see figure opposite). The maximum wavelength depends on the amino acid sequence composition of the protein from opsin.
The ommatidies are characterized according to the presence of certain types of receptors:
- Type I : 1 UV and blue receptor
- Type II : 2 UV receptors
- Type III : 2 blue receptors
The ancestral photoreceptor in Ptérygotes (winged insects) seems to be sensitive to UV, blue and green. These types of receptors are found in a large proportion of ommatidies. Some species have 6 different green receptors. Thus, hymenoptera (wasps, bees and bumblebees) and lepidoptera of the genera Vanessa or Mandura are qualified as chromatic sorting: the ability to perceive 3 colours.
In diptera, there are two other types of ommatidies : “pale” and “yellow”, composed of 6 receptors (R1 to R6) covering the spectrum from 300 to 600 nm which corresponds to a combination of opsins and pigments sensitive and absorbent UV.
In many insects, the proportion of different visual pigments varies depending on the function that vision plays. For example, in bees, the ommatidies in the dorsal position are sensitive mainly to UV and blue, while those in the ventral position are sensitive to green. This configuration is found in many flying species: the eye is actually formed to balance the insect during flight by maintaining the horizon in the center of vision, sky (blue) and ground (green). There are also gender variations. In the butterfly Lycaena rubidus, females have sensitive dorsal ommatidies at wavelengths of 568 nm, absent in males.
Many species of lepidoptera, odonates (dragonflies) and possibly some beetles and hymenoptera have more than 3 types of color-sensitive photoreceptors with for example maximum sensitivity for red or purple. This perception is made possible by an increase in the diversity of opsin types (gene duplication for example) or by the use of filters controlling the amount of light reaching the pigments, or even by a combination of these two strategies.
The spectral diversity of visual reception can be ensured within photoreceptor cells in two ways : 1) Have the same opsin molecule and 3 different pigment filters surrounding the rhabdomere, 2) Have 5 different opsin molecules and a peri-habdomal filter, as in the butterfly Papilio xuthus.
Red sensitivity is obtained by the presence of opine sensitive at wavelengths close to 575 nm, the same sensitivity as human receptors (565 nm). Generally, the sensitivity of a receiver to a color is directly related to a type of opsin.
As in diptera, photoreceptors of P. xuthus are arranged in layers so that the light reaching the photoreceptor cells of the lower layers is filtered by those of the upper layers. In other insects, the lens is coloured so that the rhabdomeres receive monochromatic filtered light (consisting of a single wavelength). For example, many species of flies have vertical or horizontal lines of varying colours on the surface of their eyes: male of the family Dolichopodidae, flies of the family Tabanidae (horseflies) or Hermetia illucens (Stratiomyidae) (see photos below). Thus, ommatidies of the same compound eye receiving the same light profile will be stimulated differently. This mechanism allows polychromatic vision while having only one type of opsin in other words, identical photoreceptors can generate different spectral sensitivities.
Perceiving one’s environment in color offers the possibility to interact in a more complex way with it : sexual partner search, phototaxy (movement according to light), oviposition (laying behaviour), predator detection… For example, species of hymenoptera, lepidoptera or diptera may have been trained to associate colors with behaviours (video below). Also, it has been shown in bees and wasps that insects also have the ability to discriminate a shape not its color.
Many insect species favour certain colours in the search for food, this behaviour can be modified by learning and experience. For example, phytophagous insects are attracted not only by green light sources, but also by yellow sources. Bees are attracted to violet to blue light sources, an evolving response to the fact that flowers reflecting such colours contain more nectar.
IV. Vibration plane discrimination (polarization sensitivity)
Light waves vibrate in a plane perpendicular to the axis in which they move (illustration 5 below). These vibration planes can be distributed over the 360° surrounding the steering axis. When all light waves vibrate in a specific plane, the light is said to be polarized.
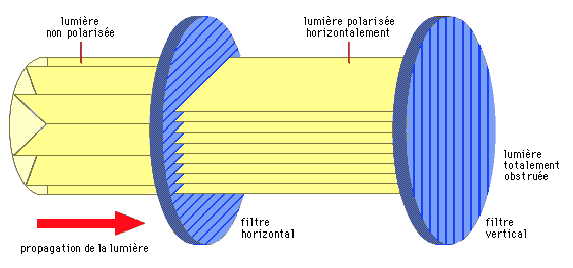
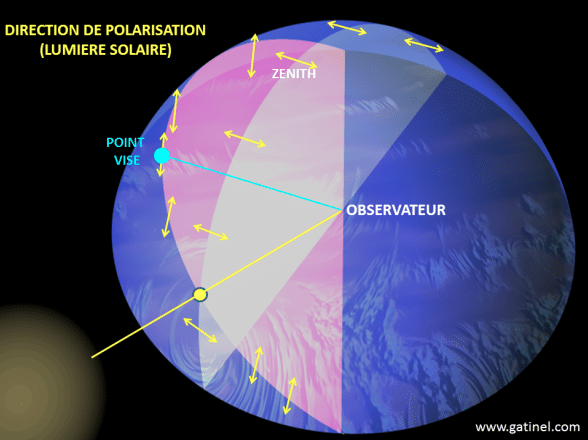
For example, blue light from the sky is polarized : the degree of polarization and the plane of maximum polarization depend on the position of the sun, and are therefore not the same in different parts of the sky (illustration 6 opposite). The detection of these parameters makes it possible to determine the position of the sun, even when it is not visible. Some insects use this information to direct themselves, including social insects such as bees (genus Apis) or ants of the genus Cataglyphis that search for food and then return to the colony. In insects such as Odonata (dragonflies) and Diptera (flies), the ability to perceive polarized light keeps navigation stable and constant.
The perception of the polarization plane is possible thanks to the presence of photosensitive pigment molecules oriented in a specific axis and located in the microvilli of the rhabdomères. Activation occurs (maximum absorption) when light vibrates in the same plane as these molecules ( Figure 7 below).
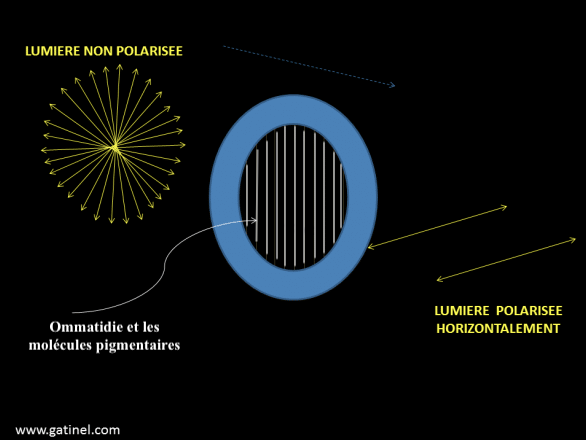
In ommatidies, the rhabdomere is most often curved (convex shape of the compound eye), so there can be no plane, no specific absorption. The polarization sensitivity will therefore depend on a uniform orientation of the visual pigment molecules in a rhabdomere. In ants and bees, as well as other polarization-sensitive insects, straight rhabdomeres are present only in a small group of ommatidies along the dorsal edge of each eye. These ommatidies constitute hardly 6% of the total ommatidies in ants Cataglyphis and 2.5% in bees of the genus Apis. The stimulation of photoreceptor cells takes place when a majority of pigment molecules are parallel to the polarization plane.
The functioning of this system is still incomplete. The hypothesis suggests that by scanning the sky, the insect is able to match these polarization receptors to the polarization planes of the sky. When redirecting, the sky pattern would match the stored entry. A mental map that could make this kind of comparison was located in the central body of the locust locust brain.
Source :
– The Insects : Structure & Function (5th edition, 2013) – Edition : S.J. Simpson & A.E. Douglas – Cambridge University Press
– Encyclopedia of Insects : Vincent H. RESH & Ring T. CARDE, Academic Press-2009
– Tibbetts E. A. & Izzo A. (2010) : Social punishment of dishonest signalers caused by mismatch between signal and behavior – Current Biology, 20(18):1637-1640 (lien)
Recommendation of books on this theme
– Evolution of the Insects(David Grimaldi & ; Michael S. Engel – Edition : Cambridge University Press – 772 pages – 16 mai 2005)
>.
– Les insectes : An Outline of Entomology (P.J. Gullan & ; P.S. Cranston – Edition : Wiley-Blackwell – 624 pages – 5ème édition : 6 août 2014)
/p>.
– Les insectes : Structure et fonction (R.F. Chapman & ; Stephen J. Simpson – Edition : Cambridge University Press – 959 pages – 5ème édition : 12 novembre 2012)em>/p>>>.
– Encyclopédie des insectes (Vincent H. Resh & ; Ring T. Cardé – Edition : Academic Press – 1024 pages – 2ème édition : 22 juillet 2009)
.